This article was previously published in LinkedIn by our Senior Sustainability Specialist Salla-Mari Marttinen
With the help of FfE, the nonprofit German Research Center for Energy Economics, my team at Hycamite carried out a life cycle assessment (LCA) of Hycamite’s hydrogen. Solid carbon also showed interesting results, indicating that we can implement a low-emission method for producing high-value carbon for industrial use.
FfE performed an LCA of our carbon products to evaluate the carbon footprint of the entire production process, including upstream emissions. Since FfE maintains its neutrality by participating in multiple projects with a diverse range of collaborators, we at Hycamite felt FfE would be an ideal, unbiased partner to guide us through the calculations and ensure validity of the results.
Celebrating a carbon footprint below 1 kg CO₂e/kg C
I work as a Senior Sustainability Specialist at Hycamite with other highly professional people, and we were all delighted by the results. According to the LCA, using Hycamite’s carbon products can significantly lower greenhouse gas (GHG) emissions when producing carbon that can be further processed into other carbon-containing products.
The impact category of the LCA was climate change, and the results showed Hycamite’s carbon footprint to be below 1 kg CO₂e/kg C. A comparison of the results of the LCA and the carbon footprint of different carbon products is shown in Figure 1. However, it is worth noting emissions are only Hycamite’s carbon as it comes out from the reactor. Upgrading that carbon to various carbon products needs more evaluation.
As is shown in Figure 1, Hycamite’s high-value carbon is superior to emissions data gathered internally from other industrial carbon product sources. However, according to Minviro, the actual carbon footprint of battery-grade graphite might even be 10 times higher than shown in the literature.
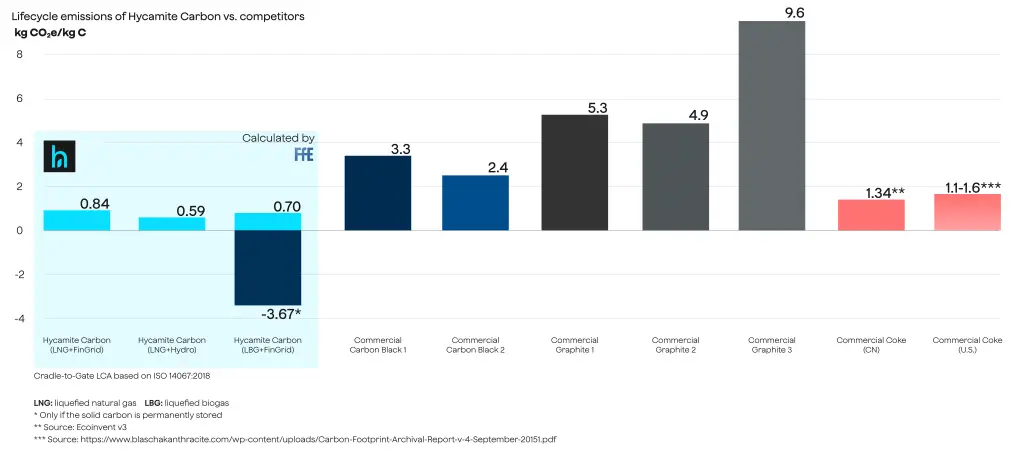
Figure 1. Life cycle emissions of different carbon products.
Evaluating the life cycle’s environmental impact
An LCA helps determine the environmental impact of products, processes and services by examining a product’s environmental effects from the extraction of raw materials to the manufacturing, use and disposal stages. LCAs can be conducted according to various system boundaries (Figure 2), such as cradle-to-gate (raw material extraction and the manufacturing processes), gate-to-gate (the manufacturing processes alone) and cradle-to-grave (entire life cycle from raw material extraction to disposal). In this instance, the LCA was based on a cradle-to-gate model, though it is still essential to map the effects to the grave.
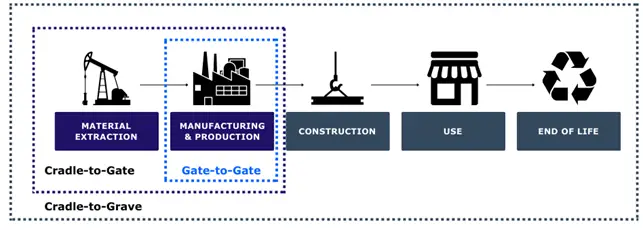
Figure 2. LCA System Boundaries
LCAs are reliable, transparent means of evaluating emissions and other environmental factors based on a standardized methodology. My previous article explains the standards and details of our LCAs for hydrogen and carbon.
Compared to the figures in my previous article, we have since made a change to the results. When we started to make more customer cases with carbon products, we realized that when sustainable biomethane is used as a feedstock, the resulting negative emissions (-3.67 kg CO₂e) should be attributed to the solid carbon, as it is physically bound to it. Doing so ensures a net neutral result in case the biogenic carbon is released back to the atmosphere as CO₂ later in the life cycle. We contacted FfE to evaluate the results, and they agreed our argument is justifiable according to the ISO 14067:2018 standard. Now, the carbon footprint of Hycamite’s hydrogen produced from biomethane will be based only on the upstream emissions (which are allocated) and solid carbon will carry all the negative emissions in the assessment plus the allocated upstream emissions. Therefore, when the carbon is sold, the party that releases the carbon is responsible for these emissions, and the hydrogen customer will not have to think about what happens to the carbon. It is important to note that there are different ways to do the allocation, so there is no right or wrong way, as long as it has been justified.
Replacing conventional carbon products increases carbon handprint
The production of solid, industrial-quality carbon distinguishes the methane-splitting method from the conventional steam methane reformation (SMR) method using carbon capture. The latter process yields carbon as CO2, which is difficult to store or reuse and is typically released into the atmosphere. Solid carbon can be stored easily and sold to manufacture items, including batteries, supercapacitors, electronic components, polymers, composite material additives and concrete. One of the carbon allotropes that could be produced via methane splitting is synthetic graphite, which the EU and the United States classify as a critical raw material.
Harnessing rather than releasing carbon reduces the net cost of hydrogen production and will further reduce GHG emissions in those industries by replacing numerous carbon-emitting products, meaning it will increase the carbon handprint. A carbon handprint is the opposite of a footprint. It recognizes the actions taken to have a positive impact on the climate, reducing one’s carbon footprint. With enough actions, a carbon handprint might even outweigh the size of one’s carbon footprint.
The methane source also impacts the products’ carbon footprint, which was considered in the sensitivity analysis. Therefore, it is imperative that the methane source produces extremely low upstream emissions, which can be achieved in part by producing hydrogen near the methane source. Consequently, using biomethane as the feedstock of methane pyrolysis is the preferred option since it may result in carbon removal from the atmosphere, depending on the biomethane’s raw materials.
Using carbon in products can be carbon negative
Climate neutrality means net-zero emissions. It is imperative that humanity prioritizes the mitigation of emissions through both avoidance and reduction strategies. Nonetheless, this cannot be reached as mankind will invariably release emissions from some activities. Consequently, to attain an overall state of carbon neutrality, we need carbon-negative technologies. Methane splitting is one such technology, as demonstrated in this article – it can indeed create carbon-negative cycles, i.e. carbon sinks.
When sourced from biogas, methane can capture carbon from a natural setting – the atmosphere – and prevent its return, thereby creating a carbon sink. A carbon sink’s effective creation depends on the biogas’ processing and feedstock. When sustainable biomethane produced close to the facility is used and the biogenic solid carbon is stored permanently in a product, the size of the carbon sink is significant. When producing 1 kg of hydrogen and 3 kg of solid carbon, the net carbon sink from the atmosphere can be as high as 11 kg CO₂. In some cases, the upstream emissions of biomethane can be negative due to avoided methane leaks to the atmosphere.
Acknowledging the importance of what happens after manufacturing
Our LCA focused on cradle-to-gate boundaries (raw material extraction and the manufacturing processes). However, when combusted, 1 kg of carbon will turn into 3.67 kg of CO₂. Therefore, what happens to the carbon after it leaves to the end customer matters. Figure 3 shows how different feedstocks behave all the way to grave.
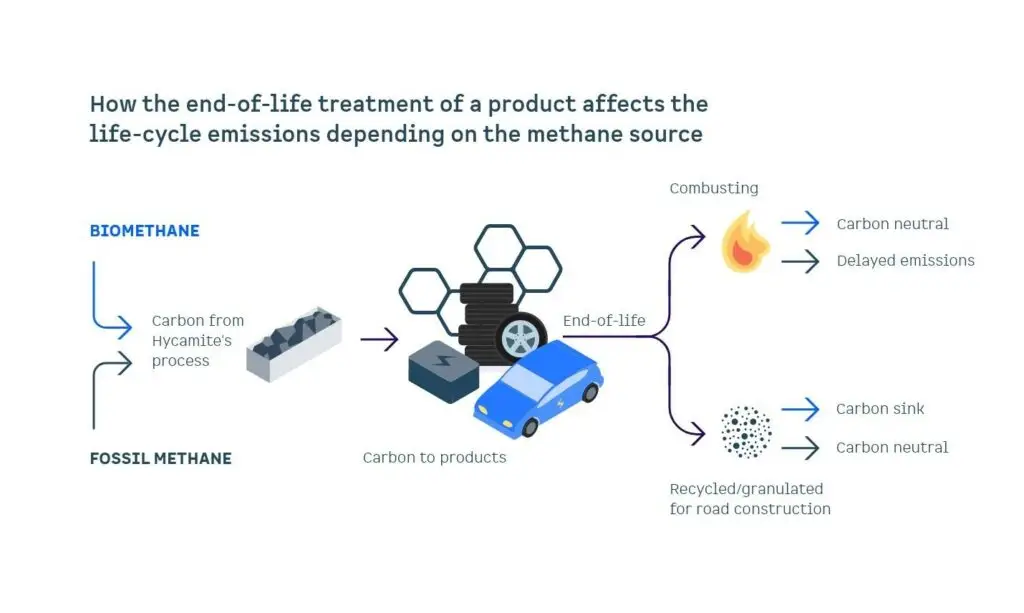
Figure 3. End-of-life treatment’s impact on life cycle emissions
At Hycamite, we favor storing carbon and aim to avoid carbon combustion. However, replacing the current emitting and harmful products with local, low-emission ones is still better than avoiding our carbon combustion altogether. If we replace emitting carbon with biogenic carbon, combustion will be net zero since that carbon was once taken from the atmosphere.
Considering other environmental impacts
In our LCA, we focused on climate change impact. However, there are also other negative environmental impacts when producing carbon with current methods, as discussed in an article by Niina Grönqvist, Hycamite’s vice president of carbon products. For instance, synthetic graphite production can seriously damage the environment without significant protection investments. Manufacturing emits nitrogen dioxide, sulfur dioxide and particulates from baking the hydrocarbon feedstocks. Earlier stages of the synthetic graphite value chain may pose serious environmental risks because of the carbon particulates, volatile organic chemicals, sulfur and heavy metals. High energy consumption can make graphite production very unsustainable. In fact, Tesla named graphite as one of the main contributors of CO₂ in their nickel-based battery supply chain – second only to nickel. All significant environmental impacts should be studied to understand the implications on a big scale – and we are here to ensure our customers can have access to sustainable products.