During the transition to green hydrogen, we need solutions for producing H2 from fossil fuels without CO2 emissions. Investments in carbon capture and storage technologies are welcome. However, we have only limited opportunities for CO2 storage or reuse. We need to develop other technologies for decarbonising hydrogen production.
To save the climate, we need to create a hydrogen economy without carbon dioxide (CO2) emissions. Hydrogen (H2) is widely produced for industrial use around the globe. However, this process still mostly involves using natural gas, methanol or coal as crude materials or source of the energy in the process. As a result, these processes create and release large amounts of CO2.
Producing green hydrogen via electrolysis using renewable energy is the ideal solution and should be the ultimate goal of the global energy system. However, building sufficient infrastructure will take a long time and immense investment. During the transition to zero-emission green hydrogen, we need solutions to the challenge of producing H2 from fossil fuels without CO2 emissions.
<h2 class="wp-block-heading" id="several-ways-to-capture-carbon-as-co<sub>2Several ways to capture carbon as CO2To that end, several carbon capture and storage (CCS) technologies are available or in development for hydrogen production. CCS refers to a suite of technologies that capture CO2 from large point sources, including power generation or industrial facilities, that use either fossil fuels or biomass for fuel.
Currently, more than 95% of hydrogen is produced by the steam-methane reforming (SMR) of natural gas. In SMR, methane (natural gas) is combined with water (steam) in high pressure using a catalyst to produce hydrogen and CO2. SMR is attractive because of its high hydrogen yield, but it also converts carbon to CO2. CCS technologies have the potential to capture 80 to 90% of CO2 emissions from the reforming process.1
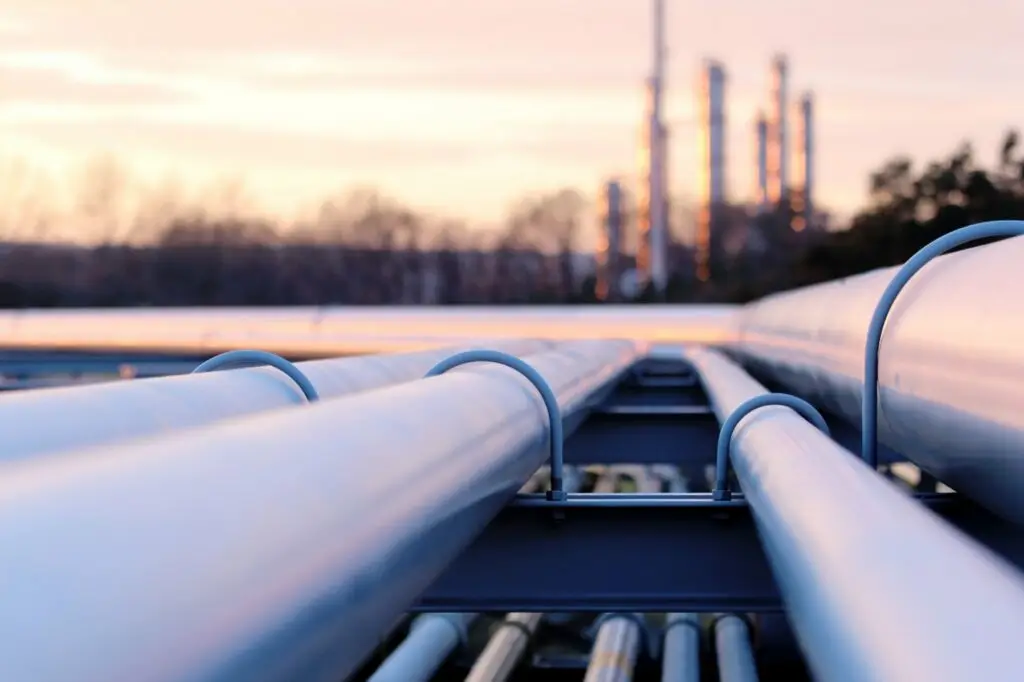
The present industry standard for SMR-based H2 plants is the capture of CO2 from shifted syngas using methyl diethanolamine (MDEA) solvent. Four other options for reducing CO2 emissions or capturing CO2 include:
- Using H2-rich burners in conjunction with CO2 capture from shifted syngas with MDEA (this method only reduces the amount of CO2 created without capturing it);
- Capturing CO2 from pressure swing adsorption (PSA) tail gas using MDEA;
- Employing cryogenic and membrane separation; and
- Capturing CO2 from flue gas using monoethanolamine (MEA).
Limited reuse opportunities
Ideally, captured carbon can be reused. However, the downside of all the technologies mentioned above is that they produce CO2 as a gas, with limited opportunities for reuse. Hydrogen production is only one of many industrial fields that plan to eliminate CO2 emissions by carbon capture, and they all compete for opportunities to place their carbon somewhere.
Methods that reuse carbon as CO2 are usually termed carbon capture, use and storage (CCUS) technologies. Many countries are increasing their support for CCUS development and deployment, including Canada, China, Japan, the Netherlands, Norway, Saudi Arabia, the UK and the USA. According to the IEA, two large-scale CCUS power projects are currently in operation with a combined CO2 capture capacity of 2.4 million tonnes per annum (mtpa).2
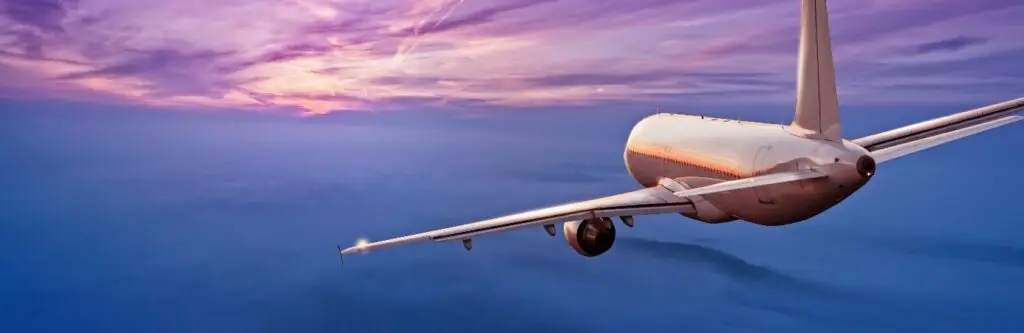
CO2 can be converted to products such as methanol, biofuel, and other hydrocarbons for use as alternative and renewable sources of energy. In fact, CO2 can technically be converted into virtually any type of fuel that is traditionally derived from petroleum. For instance, Liquid Wind in Sweden has announced its first commercial-scale electro-fuel facility that will combine captured carbon emissions with renewable hydrogen to create carbon-neutral fuel for the shipping industry.3
These synthetic fuels can play a significant role in lowering the carbon emissions from traffic. If we recycle carbon from industrial sites or power generation in transport, we cut the total CO2 emissions to half of the original. This deduction can be achieved with minimal changes in the existing infrastructure and can therefore reduce significantly and rapidly overall carbon emissions.
However, it is essential to pay attention to the energy balance in the fuel production. The additional processing of new fuel products should not require more energy than is released from burning the fuel, as the process will then require more fuel overall. The parasitic load of the CCS compressors, dryers and CO2 absorption plant typically requires 15–30% more fuel.4
Other forms of CCUS convert CO2 into commercial products such as plastics, concrete and reactants for various chemical syntheses. These products include polycarbonates, acetic acid and urea.5 However, energy use is also an area of concern for these applications. CO2 is a highly inert molecule. As a result, transforming the captured gas into industrial products typically requires a lot of energy.6
Carbon mineralization is also a useful way to reuse carbon. Carbon dioxide can be made to react with minerals such as magnesium oxide and calcium oxide to form stable solid carbonates. These carbonates can then be used for construction and consumer products. When producing cement, carbon curing – infusing wet concrete with carbon dioxide – can produce concrete that is 4 per cent carbon dioxide by mass. Carbon curing can shorten curing times and increase concrete’s water resistance and strength.7
Until now, the vast majority of CCUS projects have relied on revenue from the sale of CO2 to oil companies for enhanced oil recovery (EOR). In this process, captured CO2 is injected into depleted oil fields to increase the amount of oil that can be extracted from the wells.
However, there are limits to reusing carbon from CO2 with all these techniques mentioned to this point. For instance, the total quantity of carbon dioxide that might eventually produce chemicals, plastics and carbon fibre would be too small – between 40 million and 90 million metric tons per year – to make an appreciable dent in global greenhouse gas emissions.8 Fuel production, concrete enrichment and power generation could reduce annual greenhouse gas emissions by as much as one billion metric tons in 2030.9 Although these are significant reductions, global CO2 emissions have increased from 2 billion tonnes of carbon dioxide in 1900 to over 36 billion tonnes 115 years later.10
Where should all that CO2 be stored?
If captured carbon cannot be reused, it must be stored somewhere.
The Global CCS Institute, an international think tank, publishes a database of some 60 CCS facilities in various stages of development with a combined CO2 capture capacity of more than 127 mtpa. There are now over 20 facilities in operation, three under construction, and 35 in various stages of development.11
One of the most interesting projects in the database is in the UK. Drax Bioenergy aims to use CCS to capture 4 mtpa from one of the existing biomass-fired power units by 2027; it then plans to convert all of its remaining biomass units to bioenergy with carbon capture and storage (BECCS or Bio-CCS) by 2035. The CO2 will be transported by pipeline and stored in the southern North Sea via dedicated geological storage. The project will be an anchor for the wider Zero Carbon Humber Cluster. In short, BECCS technology makes it possible to create carbon sinks.12
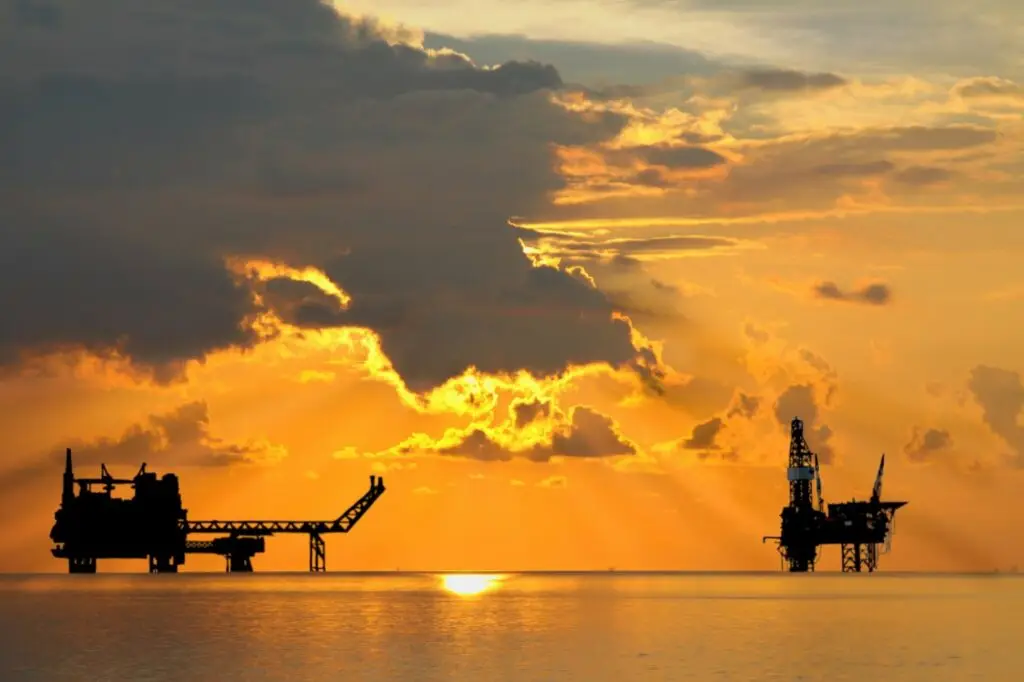
In Norway, the government has launched the Longship project for carbon capture and storage.13 Carbon capture will be implemented at Norcem’s cement factory in Brevik and at Fortum Oslo Varme waste-to-energy capture plant. The liquid CO2 will be transported from Brevik and Oslo to a terminal on Norway’s west coast and from there, CO2 will be pumped through pipelines to a reservoir beneath the sea bottom. Equinor and its partners, Shell and Total, are responsible for planning the storage facility.14
Both of these projects are based on storing CO2 below the sea, a few kilometres under the seabed. Reusing old offshore platforms is one of the most promising ways to store captured greenhouse gases. Many of the methods and processes already used in the gas industry could be modified and adapted to the specific requirements of CO2 storage. However, the problem is the required scale.
The Potsdam Institute for Climate Impact Research has been evaluating available CO2 storage options in Germany. In the country, the storage volume of extracted gas fields is significantly lower compared to other options. With an energy-related CO2 emission rate in Germany of 865 mtpa or 393 mtpa (only industrial, punctiform CO2 sources > 100,000 per year) in 2005, old gas fields would be filled in a few years.15
Deep saline aquifers are estimated to have by far the largest capacity in Germany. However, these estimates can only be realized in the medium to long term, and much more research is needed. This option, as well as storing the gas in deep coal seams, poses a latent risk of leaking, which can occur in the geological formation as well as in pipeline or other transport systems. The costs for storage in saline aquifers are estimated to be relatively high, as the usable aquifers are located at a depth of one kilometre, and existing boreholes can only rarely be used to introduce CO2. Furthermore, the water reservoirs in these structures must first be displaced using suitable injection strategies. Overall, the method is technically feasible but requires significantly more effort than storage in gas and oil fields.
CCS technology is still under development
Potential CO2 leaks from underground sinks are not the only problems with CCS. CCS technology is still under development. Pilot plants have shown that CCS is technically possible, but the costs are still high.
According to the Global CCS Institute’s report from 2017, the cost of avoided CO2 emissions ranges from USD 21.5/tonne for gas processing and bio-ethanol production, around USD 78/tonne for coal-fired power generation, USD 89/tonne for gas-fired power generation and up to USC 124/tonne for cement production.16
All in all, investments in CCS technologies are welcome and necessary. We need CCS alongside other technologies for mitigating greenhouse gases before global temperatures rise too far. However, CCS will not be a magic-wand solution that solves all or even most of our problems with CO2. We need other technologies, especially for hydrogen production. In practice, some other sectors than hydrogen production will simply not be able to achieve net-zero emissions without CCUS,17 and hydrogen production must compete with CO2 storage and reuse options. I will discuss other opportunities to avoid CO2 emissions in hydrogen production in my next article.
Sources:
1) http://ieahydrogen.org/pdfs/TechnologyRoadmapHydrogenandFuelCells-(1).aspx
2) https://www.iea.org/reports/ccus-in-power
3) https://www.liquidwind.se/news/ovikenergi
4) https://www.energyvoice.com/opinion/195477/ccus-is-a-stopgap-to-a-big-hydrogen-world/
5) https://www.nap.edu/catalog/10153/carbon-management-implications-for-rd-in-the-chemical-sciences-and
6) https://www.mckinsey.com/business-functions/sustainability/our-insights/why-commercial-use-could-be-the-future-of-carbon-capture
7) https://www.mckinsey.com/business-functions/sustainability/our-insights/why-commercial-use-could-be-the-future-of-carbon-capture
8) https://www.mckinsey.com/business-functions/sustainability/our-insights/why-commercial-use-could-be-the-future-of-carbon-capture
9) https://www.mckinsey.com/business-functions/sustainability/our-insights/why-commercial-use-could-be-the-future-of-carbon-capture
10) https://ourworldindata.org/co2-and-other-greenhouse-gas-emissions#how-have-global-co2-emissions-changed-over-time
11) https://co2re.co/FacilityData
12) https://www.globalccsinstitute.com/news-media/press-room/media-releases/
13) https://www.regjeringen.no/en/aktuelt/the-government-launches-longship-for-carbon-capture-and-storage-in-norway/id2765288/
14) https://www.fortum.com/media/2018/11/full-scale-carbon-capture-and-storage-ccs-project-initiated-norway
15) https://www.pik-potsdam.de/members/edenh/publications-1/reccs-long-version
16) https://www.globalccsinstitute.com/archive/hub/publications/201688/global-ccs-cost-updatev4.pdf
17) https://www.iea.org/reports/ccus-in-clean-energy-transitions/a-new-era-for-ccus#growing-ccus-momentum